The Environmental Protection Agency (EPA) on April 10 announ...
Read More Automating New EPA PFAS Drinking Water Testing Workflow to Maximize Lab Productivity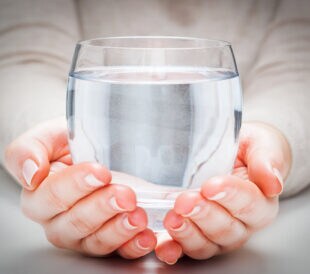
Your educational resource for biopharma, pharma, environmental, food and agriculture, industrial, and clinical labs
Your educational resource for biopharma, pharma, environmental, food and agriculture, industrial, and clinical labs
The Environmental Protection Agency (EPA) on April 10 announ...
Read More Automating New EPA PFAS Drinking Water Testing Workflow to Maximize Lab ProductivityHappy Earth Day! Since its first celebration on April 22, 19...
Read More Enabling Science, Industry to Protect the PlanetStrontium has 4 isotopes, 84Sr, 86Sr, 87Sr and 88Sr. One of ...
Read More How to Minimize Sample Preparation and Optimize Sr Isotope Ratio AnalysisI used to joke in graduate school that how fast I could actu...
Read More Tired of HPLC Downtime? Time for a Technology Boost?Chlorinated dioxins and furans Dioxins are a collective term...
Read More What are Dioxins and PCBs, and What Type of GC-MS Should You Use to Analyze Them?This is part two of a three-part series on Thermo Fisher Sci...
Read More Advanced LC-MS Tools and Software Solutions to Ease Your Metabolite Identification ChallengesThis is the fifth in a five-part BioPharma Blog Series. Have...
Read More Unveiling Hidden Impurities: LC-HRMS for Biopharmaceutical AnalysisWater is a precious resource that is vital for all aspects o...
Read More Practical Analysis Solutions to Enhance Water Quality for a Safer FutureIt’s World Water Day, but do we truly understand the impor...
Read More World Water Day: A Good Time to Reflect on the Critical Role of WaterIn an era defined by environmental consciousness and operati...
Read More Water Management for Industrial and Chemical Manufacturers to Enhance Sustainability and Efficiency